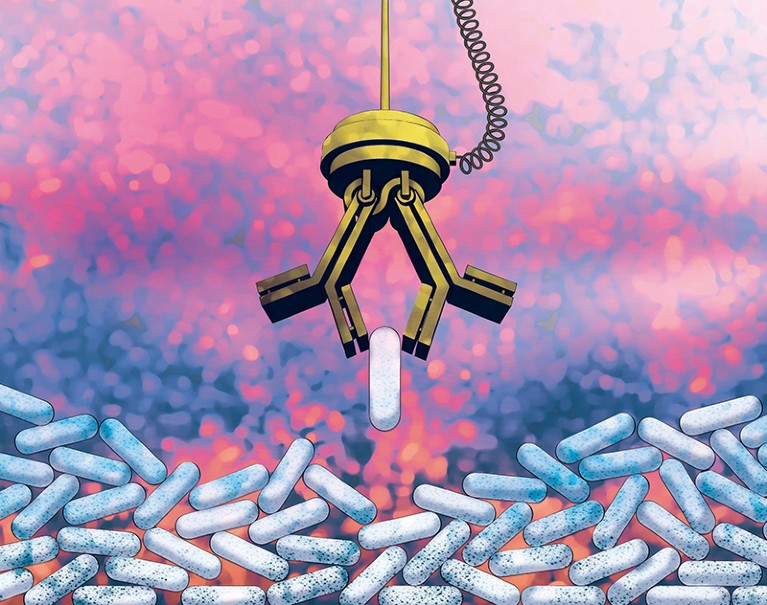
Credit: S. Bradbrook / Springer Nature Limited
The relationship between the immune system and microorganisms was long viewed as a war rather than a union, as it was mostly studied in the context of host defence against pathogens. Thus, when deficiency in lymphoid organ development and immune cell activity was reported in germ-free animals in the 1960s, this first evidence that microbiota shape immune homeostasis was interpreted as education of the immune system by infections.
This concept laid the foundation for the ‘hygiene hypothesis’, formalized by David Strachan in 1989 in his influential study reporting lower incidence of hay fever and eczema in children with older siblings. He proposed that infections in early childhood prevent atopy later in life, and that increased allergy prevalence in developed countries may be caused by high standards of personal hygiene. Further developments of the hygiene hypothesis correlated pathogen exposure to decreased allergy risk in humans, and established a role for microbiota in oral tolerance in mice, presumably in shifting an immune set point from T helper 2 (Th2) to Th1 cell responses.
In parallel, fundamental principles of microorganism recognition by the immune system unfolded. In 1989, Charles Janeway proposed that immune responses are initiated by genome-encoded pattern recognition receptors (PRR) on immune cells, which sense conserved microbial molecules. Over the next decade, PRRs specific to various bacterial, fungal and viral components were discovered. However, it became evident that PRRs are not specific to pathogens, posing the question of how commensals, which colonize mucosal surfaces, coexist with the immune system.
The prevailing explanation was that commensals and immune cells are separated by epithelial barriers. This view was challenged in the early 2000s by accumulating examples of immune responses to commensals in healthy mice. Among these, Andrew Macpherson, Rolf Zinkernagel and colleagues found that in mice, commensals are recognized and compartmentalized by gut lumen-secreted IgA. Lora Hooper, Jeffrey Gordon and coworkers reported that a commensal bacterium stimulates antimicrobial peptide production by Paneth cells.
In 2004, Seth Rakoff-Nahoum and Ruslan Medzhitov provided evidence that the immune system senses commensals through PRRs under normal conditions and that this sensing is crucial for tissue repair. This finding opened a new perspective on immune response to microorganisms not as host defence, but as a symbiotic physiological process.
Realization of beneficial roles of immune–microbial interactions prompted a revision of the hygiene hypothesis to postulate that protection from allergic diseases is mediated by early-life exposure to commensals rather than pathogens (Milestone 5). The refined hypothesis suggested that the rise of allergies in industrialized societies is caused by loss of commensals. A link between lifestyle, microbiota and allergic diseases has been now confirmed in a large number of human observational studies, and expanded to implicate microbiota in other chronic inflammatory and autoimmune pathologies, as well as metabolic and neurological disease.
Dissecting the underlying cellular and molecular complexity of microbiota–immune interactions has occupied microbiologists and immunologists to this day. Pioneering works by Sarkis Mazmanian, Dennis Kasper and colleagues showed that microbiota-guided maturation of the mouse immune system can be recapitulated by a polysaccharide produced by a symbiotic bacterium, and delineated that its uptake by dendritic cells promotes antigen presentation, inducing expansion and differentiation of CD4+ T cells into Th1 and regulatory T cell lineages. Ivaylo Ivanov, Kenya Honda, Dan Littman and colleagues uncovered how specific commensal bacteria direct Th17 cell differentiation in the small intestine. In 2013–2014, four groups independently discovered a mechanism of immune tolerance mediated by metabolites of intestinal commensals (Milestone 21).
Our intimate companion in sickness and in health, microbiota impacts our physiology to a large extent through interactions with the immune system at mucosal sites. How these interactions, ranging from host defence to active tolerance to symbiosis, integrate into physiological outcomes remains an exciting avenue to explore.