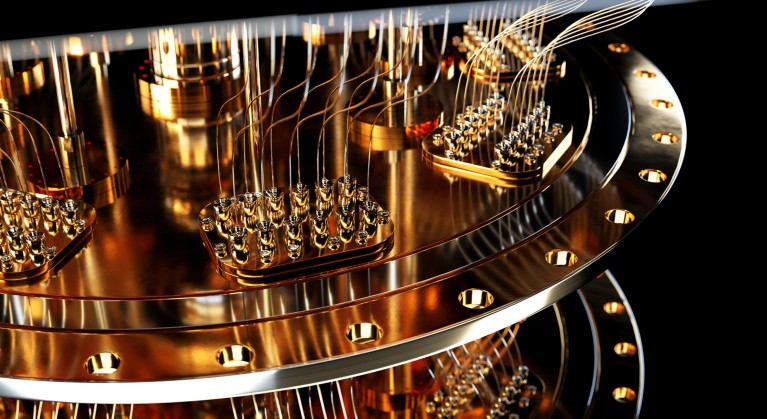
A section of a quantum computer based on superconductors. Researchers at NEC are exploring such quantum computers. Credit: Bartlomiej Wroblewski/iSatock/Getty
“These computers will drastically change the world,” predicts Tsuyoshi Yamamoto, a physicist with NEC Corporation, headquartered in Tokyo, Japan. He is referring to the promise of quantum computers — machines that exploit the laws of physics governing the atomic and subatomic realm.
Quantum computers are expected to outperform even the most powerful conventional supercomputers. In fact, one day quantum computers will be able to zip through problems so complex that it would take our current best machines longer than the age of the Universe to solve, physicists predict.
“A completely new breed of computer, quantum computers are expected to solve problems that are intractable with conventional computers — even supercomputers,” explains Yamamoto, who is spearheading NEC’s ambitious quantum-computing programme. “For instance, quantum computers could allow us to simulate the complexity of chemical reactions and to design new materials and drugs.”
The global race to build a practical quantum computer has been accelerating over the past decade. In 2019, Japan launched its 100 billion yen (US$668 million) Moonshot R&D Program, with one of its key goals being to create such a computer by 2050.
Now, NEC Corporation is bringing together multiple research teams at a number of universities and laboratories in Japan with the expertise needed to make this vision a reality. In particular, they are using qubits — the quantum equivalent of bits in conventional computers — based on superconductors to make quantum computers. Together, they are using a multi-pronged approach to tackle some of the biggest challenges currently hampering the field.
Useful quantum computers
One of the biggest hurdles is scalability — creating quantum computers that are large enough to tackle real-life problems. Of the five or so platforms being pursued in the race to develop quantum computers, superconductors is one of the platforms currently out in front. In 2023, a team at IBM succeeded in connecting more than 1,000 superconducting qubits.
Such rudimentary machines can outperform standard computers at highly specific, yet esoteric tasks. “But those applications have yet to find a practical use,” notes Yamamoto.
The trouble is that no reliable way currently exists to correct for errors introduced during calculations. So to create a quantum computer that can tackle useful problems, physicists either need a lot more qubits — to overwhelm occasional errors — or they need to find a good way to reduce errors.
“If an error-correction scheme is implemented, it is theoretically established that quantum computers will be able to perform some useful computations much faster than classical computers,” says Yamamoto. “However, to run an algorithm with an error-correction scheme, we need a tremendously large number of qubits — 100 million according to one estimate.”
Two-prong approach
The project is approaching the challenge from both sides: by increasing the manageable number of qubits and by decreasing the number of qubits required.
Their hardware employs circuits built from superconductors — materials that allow electricity to flow through without any resistance when they are chilled to ultralow temperatures. Each qubit consists of a tiny superconducting circuit. Information is encoded in the energy of these mini-circuits: the two lowest possible energy states correspond to the values of 0 and 1.
Fabricating many thousands of qubits on a wafer may not be so difficult, notes Yamamoto. The hard part comes in trying to communicate with each of those qubits in a refrigerator. Each qubit must be connected to wires so that physicists can send in electrical signals to control the qubit and encode information, and also read out the state of the qubit during and after performing a calculation.
Squishing all of those wires into a small space is difficult and likely to degrade the qubits. The problem is exacerbated because superconducting qubits are controlled by microwaves transmitted along metallic coaxial cables, which carry heat that can warm up the refrigerator and the qubits.
To tackle these wiring problems, Masamitsu Tanaka, a physicist at Nagoya University, in Japan, and his colleagues are developing innovative ‘cryo-electronics’ — electronics for qubit control and readout that operate at cryogenic temperatures near the qubits. “That will naturally reduce the hardware needed to go between room-temperature electronics to the qubits,” says Tanaka.
To date, they have demonstrated that their cryo-electronics can operate at ultrafast clock frequencies at temperatures as low as just four degrees above absolute zero. The team is now working on reducing the energy consumption to minimize the heat generated next to the qubits1.
Bosonic codes
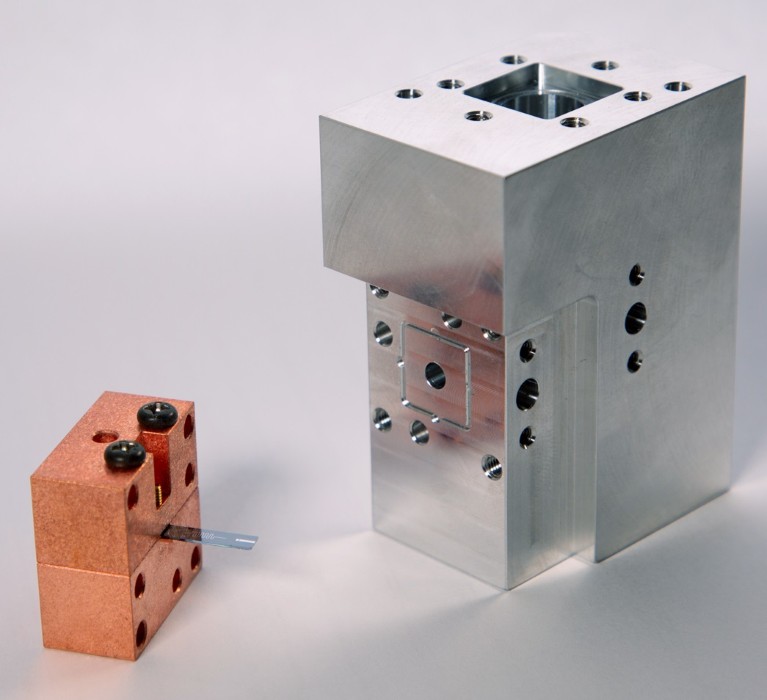
An aluminum cavity and an ancilla qubit chip (sandwiched in a copper block) developed by Shiro Saito’s lab at NTT to implement a bosonic qubit.
Meanwhile, physicist Shiro Saito of the NTT Basic Research Laboratories in Atsugi, Kanagawa, Japan, is working on ways to correct errors in processing2. The energy levels of a qubit can be pictured like a ladder with two rungs. If a qubit is disturbed, its value could easily flip between 0 (which corresponds to the lowest step) and 1 (usually the second step), without anyone realizing. To correct this error, the qubit information must be encoded into many such ladders.
More than two decades ago, however, physicists proposed a ‘bosonic code’ scheme to implement a new way of error correction. The trick is to encode the qubit information in the many rungs of a full ladder, rather than many two-rung ladders. This redundant encoding allows the detection of an error while maintaining qubit information. As a result, hardware-efficient error correction can be achieved by utilizing many energy levels in a single physical system.
“Bosonic codes are very hard to implement because there are more levels that need to be controlled, and so they’re still at an early stage of research,” Saito explains. “But they are worth it because they could drastically reduce the number of qubits needed to build a working quantum computer.”
With their roadmap to meet the Moonshot goal in 2050 in hand, the NEC collaboration plans to demonstrate that each component of their strategy works over the next five years. They will then integrate them together within the next decade. And then, what’s left? “After that, we must simply work hard,” says Yamamoto.