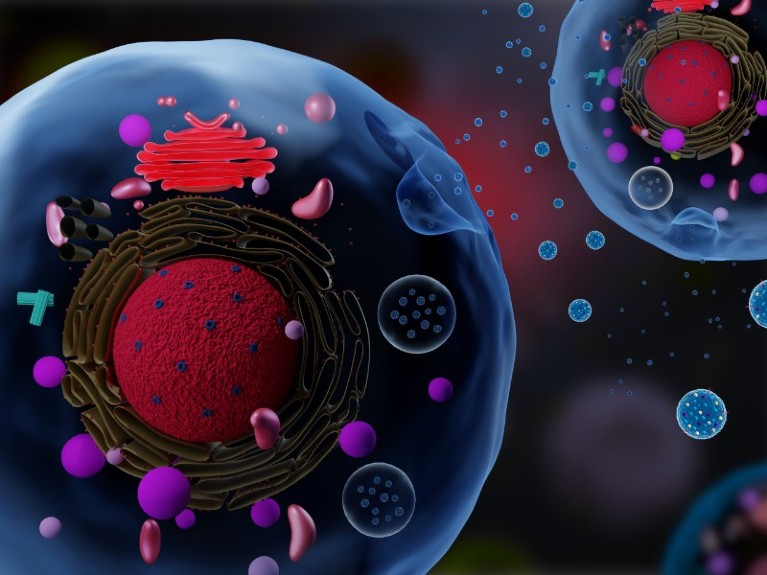
During the process of exocytosis, cells release membrane-bound vesicles (shown) into the extracellular space. Such bioparticles facilitate cell-to-cell signalling.Credit: Meletios Verras/Shutterstock
In science, some of the most valuable discoveries hide in plain sight. Such was the case for extracellular vesicles (EVs). The small lipid-bilayer compartments are released from cells and contain nucleic acids, proteins and lipids. For decades, most researchers considered them insignificant. Many referred to them simply as platelet dust1.
In 2006, a series of published papers detailed the roles of EVs in intracellular communication. The findings spurred a wave of research. Between 2010 and 2019, the published mentions of EVs grew by 10 times from around 400 to more than 50002. Researchers now believe that EVs, which can be characterized into distinct subtypes, are vital in cell-to-cell signaling, and could serve as drug-delivery vectors and disease biomarkers.
“Extracellular vesicles are a really hot area of research right now,” says Stephanie Brunelle, a molecular biologist, and senior product manager for flow cytometry at the biotechnology company, Luminex in Seattle. “Many think they could be the next big biomarker.”
The challenge, Brunelle says, is analyzing and quantifying them.
While a number of methods exist, one of the most logical ones, flow cytometry, was until recently out of reach3. Flow cytometry is a bench-standard technique for cell sorting and quantification, and lends itself to high-throughput methods. But it was not sensitive enough to assess EVs or any other small bioparticles, whether artificial nanoparticles or small bacterial cells. Advances in imaging, assays and software are now enabling small-particle flow cytometry, and will almost certainly drive an even bigger wave of published EV research in the years ahead.
A better flow
Researchers have long used flow cytometry to count and characterize cells as microfluidics guide them over a detector. But human cells can be relatively large, up to 150 micrometres in diameter. EVs are decidedly smaller. One particularly interesting subtype of EVs, exosomes, have diameters between 30 to 100 nanometres, three orders of magnitude smaller than the average cell4.
Particles that small often emit signals too dim for standard flow cytometers to reliably detect, pushing researchers and companies to improve them. For example, flow cytometers traditionally used a photomultiplier tube as a sensor, but more modern devices incorporate more sensitive avalanche photodiodes or even CCD cameras, which can be five to 10 times as sensitive as PMTs. Luminex, for instance, makes a camera-based system.
“This technology is really great for detecting small particles,” Brunelle says.
Likewise researchers have developed improved assays and detection algorithms and their efforts have made the latest flow cytometers and techniques well suited to analysing and quantifying bioparticles.
Work is now ongoing to detect even dimmer signals. Many researchers are now interested in specific molecules inside EVs or carried on their surface, which can yield important clues about EVs’ purpose and mechanisms. But signals from those molecules can be between 10,000 and one million times dimmer than standard cells.
“This is the crux of why it's been hard to apply flow cytometry, which works so well in cells, for these small particles,” says John Nolan, a biochemist at the Scintillon Institute, a research organization in San Diego, California, and CEO of Cellarcus Biosciences. At Cellarcus, Nolan and others have developed a membrane stain that causes EVs to fluoresce brightly enough for a camera to detect5. The company also uses fluorescent-tagged antibodies, which they validate to make sure they’re selectively binding to the desired surface molecules.
While researchers could develop similar tools themselves, the availability of a simple kit can be a force multiplier for research. “It’s a hard measurement to make, and you have to do about a dozen things correctly,” Nolan says. “You don’t want it to be a physics project. You want it to be a clinical test at some point.”
The right signal
Gains in the sensitivity of flow cytometry are welcome, but they also can increase noise, whether from debris in the sample, autofluorescence in the buffer, or other factors. Researchers need to manage that risk to get reproducible results.
Perhaps the most important consideration, Brunelle says, is to run controls to calibrate the equipment, as well as to validate the EV sample. Researchers need to look at the buffer solution first by itself and then with fluorescent stain or antibodies added in order to calibrate their equipment. That way, when they make measurements on actual samples, those will be comparable to measurements made at a different time or on a different sample.
Likewise, Brunelle recommends performing incremental dilutions on a sample. By gradually reducing the concentration, researchers can determine which mixtures emit too much signal, saturating the detector, and which yield too little to be seen.
Using established standards and protocols is also important. Because small particle flow cytometry is still new, not all the standards have been set. But the International Society for Extracellular Vesicles publishes a series of guidelines laying out the controls and protocols scientists should follow to make sure they have a well validated particle population.
“Not all researchers are aware of this because EV research is still a little bit like the wild west, where people are kind of doing whatever they want,” Brunelle says. “But how can you be so sure that what you're seeing is true and real without using all the proper controls, especially something that's so technically challenging because it's so small?” Software, too, can help reduce noise by picking out weak signals. Luminex has an algorithm that can determine that a dim streak across the field of view of the camera is a signal from a single particle moving across the detector. It will then integrate that into a stronger signal.
More work remains. Nolan acknowledges that some of the smallest particles are still at the edge of reliable detection for flow cytometry. Also, researchers have found a surprising heterogeneity in EVs. It would be useful to sort small particles into different subgroups as they pass through the flow cytometer, as is done commonly with cell types. That could help researchers pair their work with further analysis, such as mass spectrometry. One possibility, Nolan says, could be to attach magnetic beads to antibodies, but those would then need to be removed somehow, and unlike cells, which can proliferate after sorting, it’s not clear how to get a large enough volume of EVs.
Almost certainly, these incremental improvements will come. “This is building on 20, 25 years of quantitative flow cytometry, and these concepts are well established for quantitative cell analysis,” Nolan says. “We are largely just adapting it down to this new, dim regime.”