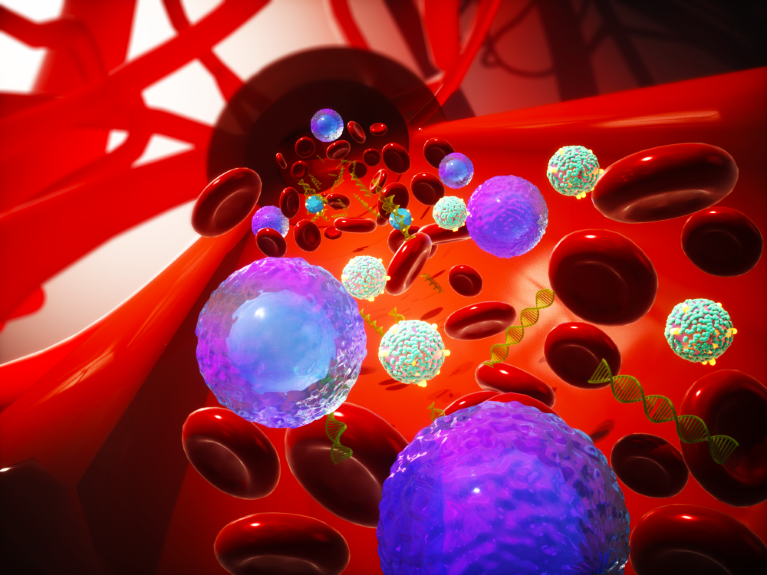
Alongside the red and white blood cells, the bloodstream contains a host of other molecules that can provide researchers with valuable information about cancer.Credit: Ella Maru Studio
“We’ve known for many years that there is tumour-related material in the blood stream,” says Minetta C. Liu. “We just didn’t have the technologies to detect it with enough sensitivity for it to be meaningful.”
Liu, a medical oncologist and cancer investigator at the Mayo Clinic in Rochester, Minnesota, is one of a growing number of researchers advancing liquid biopsies as tools to understand cancer biology. The analyses of biomarkers in body fluids offers a minimally invasive and easily repeatable way to detect cancer-associated changes in the genome, epigenome, transcriptome and proteome. The wealth of information that can be obtained from material in blood and urine is opening new avenues for both research and disease monitoring.
In recent years, investigators have greatly improved the detection of many cancer biomarkers in liquid biopsies. Tests based on these biomarkers could, one day, do more than simply identify the presence of a tumour. They could help identify its location, stage, progression, and response to therapies, representing a paradigm shift in cancer diagnosis and management.
In research, the saying goes, extraordinary claims require extraordinary evidence. Where are those working on liquid biopsies placing their focus?
State of the technology
The current generation of approved liquid biopsies are simple. Blood samples are analysed for specific proteins, namely prostate-specific antigen (PSA) and carcinoembryonic antigen (CEA), which are clear cancer signals. However, existing tests prioritize sensitivity — the ability to detect these proteins — so they tend to have high false-positive rates. This reduced specificity can lead to over-diagnosis and overtreatment, which can be harmful.
Next-generation sequencing (NGS) technologies are addressing these shortcomings and could fulfil the promise of personalized medicine as researchers obtain omic-level data on tumour material. Advances in NGS are leading to marked improvements in the accuracy and detection limit of liquid biopsies and the potential number of measurable biomarkers per assay. “Applying omic technologies to develop blood-based biomarkers will further expand opportunities in the research and clinical spaces,” Liu says.
Biomarkers include circulating tumour cells (CTCs), shed from fast-growing or late-stage tumours, extracellular vesicles or exosomes, and circulating cell-free nucleic acids (cfDNA and cfRNA). Researchers are actively evaluating the information that each of these can provide about carcinogenesis.
“It’s not just about detection, but obtaining information about a tumour that could eventually guide treatment decisions,” Liu says.
Some now argue that more information can be gained about a tumour from a blood sample than a tissue biopsy. A tissue biopsy offers a snapshot of a particular section of a tumour at a given time. Liquid biopsies can capture the heterogeneity of a tumour by providing a composite of genetic and epigenetic alterations across distinct tumour-cell subpopulations.
“cfDNA captures both clonal and subclonal mutations and thus provides a comprehensive picture of a patient’s cancer,” says Jasmine Zhou, a cancer screening specialist at the Department of Pathology and Laboratory Medicine in the David Geffen School of Medicine, UCLA.
Blood samples can also be taken repeatedly, allowing researchers to track tumours as they develop and change. “Serial assessments of a cancer over time will lead to new insights about the underlying biologic mechanisms of recurrence, drug resistance and metastasis,” Liu says.
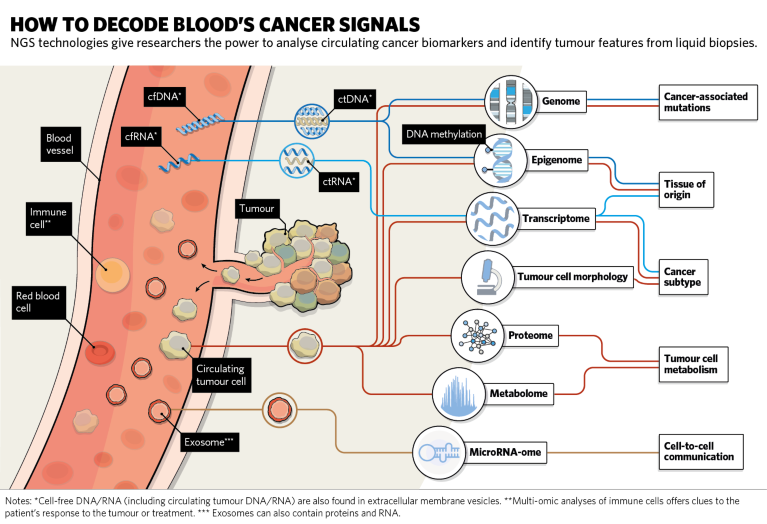
Pushing the limits of DNA detection
Of all the research into liquid biopsies, much has focused on the detection of circulating cfDNA. Most cfDNA is shed from dying blood cells, but in people with cancer there could also be detectable circulating tumour DNA (ctDNA). If the tumour is small and not metastatic, ctDNA are typically extremely low, below 10 parts per million within cfDNA. Aadel Chaudhuri, a physician-scientist at Washington University in St. Louis who specializes in lung cancer, describes the challenge of detecting ctDNA in early stage cancer as “finding a needle in a city of haystacks”.
Even if the specificity of ctDNA detection is high, the sensitivity remains low because so little exists. To address this, Chaudhuri and others have used CAPP-Seq (CAncer Personalized Profiling by deep Sequencing). The ultrasensitive NGS-based method identifies and quantifies recurrent cancer mutations, determined from publicly available sequencing data, in patients’ cfDNA. Chaudhuri and colleagues have shown that it can accurately predict lung cancer relapse.1
“We can detect very low levels of tumour variants in plasma that are indicative of residual cancer following treatment,” Chaudhuri says. “As the assays get better, liquid biopsies could eventually become a standard method for detecting cancer relapse.”
Chaudhuri is also working on improving the detection of ctDNA in urine samples.2,3 “Urine is an even easier analyte to obtain than blood,” he explains. “High levels of tumour-derived DNA can be found in the urine of patients with bladder cancer and can be detected, at much lower levels, in patients with some nongenitourinary cancers, such as colorectal cancer.”
Close inspection of tumour-specific mutations in ctDNA could shed light on the molecular mechanisms that drive cancer cell proliferation and the development of resistance to treatment.
Beyond mutations
With improved tools and methods at their disposal, researchers are now looking beyond genetic mutations in cfDNA to new types biomarkers, each with their own utility. Chief among them are epigenetic alterations. “Epigenetic marks reflect an individual’s exposure to different environmental factors, and thus, to their real-time risk of developing cancer,” Zhou says.
cfDNA methylation profiling, in particular, seems to hold promise as an approach for early cancer detection.4 Changes in the patterns and levels of DNA methylation can distinguish cancer-tissue DNA from healthy-tissue DNA. Importantly, because DNA methylation is tissue-specific, methylation profiling can identify the source of the cancer signal.
The Californian biotechnology company Grail has recently launched a multi-cancer screening test based on the detection of methylation signatures in cfDNA, using NGS and machine-learning algorithms. Grail’s test, and other multi-cancer early detection tests in development, will not replace standard screening programmes, but they could help with early detection of many cancers not typically included. Early detection of liver or pancreatic cancers, for example, could make a real difference to patients’ lives.
Liu, whose team collaborated with Grail to develop and validate the multi-cancer test, says she is excited about how quickly the field has evolved, but she also urges caution. Though tests are now clinically available, the workflows and guidelines for how to use them are still to be developed.
Another biomarker attracting interest is cfRNA. Analysis of transcribed genes reflects activity within cancer cells more accurately than cfDNA, and could also indicate targetable pathways. Researchers at Grail recently embarked on the characterization of cfRNA of individuals with and without cancer. As part of the Circulating Cell-free Genome Atlas study (NCT02889978), they sequenced whole transcriptomes from the plasma of 46 breast cancer patients, 30 lung cancer patients, and 89 non-cancer participants. They found more than 20 transcripts that were only present in cancer plasma. Half of these cancer cfRNA were tissue-specific, and some were also specific to a certain cancer subtypes.5
These findings highlight the potential of cfRNA as a cancer biomarker. “Data are still emerging,” Chaudhuri says. “But the fact that cfRNA can be isolated and profiled suggests enormous potential for non-invasively tracking disease biology and resistance to therapy.”
Of course, blood contains many more potential signals than cell-free nucleotides. Both CTCs and tumour-derived exosomes (that encapsulate proteins, RNA and gene regulating microRNAs) are generating significant research interest. CTCs can reveal the morphology and metabolism of tumour cells, whereas exosomes can provide insight into the communication between cancer cells and the tumour microenvironment.
CTCs are rare; even in metastatic settings patients generally have fewer than 10 CTCs per millilitre of blood. They are also challenging to extract. However, as Liu’s team has shown, single-cell analysis of CTCs can guide the development of assays to aid cancer treatment selection and disease monitoring. CTCs can also be used to develop xenograft mouse models “CTC-derived mouse models will provide clues about what drugs the patient’s cancer will respond to or be resistant to,” Chaudhuri says.
The analysis of exosomes can provide a complementary view, and offer useful information about a patient’s cancer status. Specific microRNAs inside exosomes have been linked to biological processes involved in tumour progression and response to anti-cancer therapies.6 Further understanding the function of these microRNAs will improve our understanding of how tumours modulate their microenvironment and evade the immune response as they start to grow.
Towards comprehensive cancer profiling
To obtain a high-resolution snapshot of a patient’s cancer, researchers are working on multi-modal approaches and on integrating the different types of information that can be obtained from cfDNA, cfRNA, CTCs and exosomes. “I don’t think it is going to be one size fits all,” Liu says. “There are multiple ways of looking at cancer signals in the blood, and each will have a different application depending on disease type and setting.”
Chaudhuri says his group is starting to perform multi-omics to simultaneously analyse the many components of a liquid biopsy, such as cfDNA and cfRNA. Zhou’s lab is working on a computational platform that integrates multiple features from cfDNA, including cfDNA methylation, cfDNA fragment sizes and copy number.7 Although different, the approaches have the same aim: to use all the information available in biofluids to improve understanding and treatment of cancer, says Zhou. “By aggregating multiple, validated cancer signals in the blood we will get a more accurate picture of disease.”