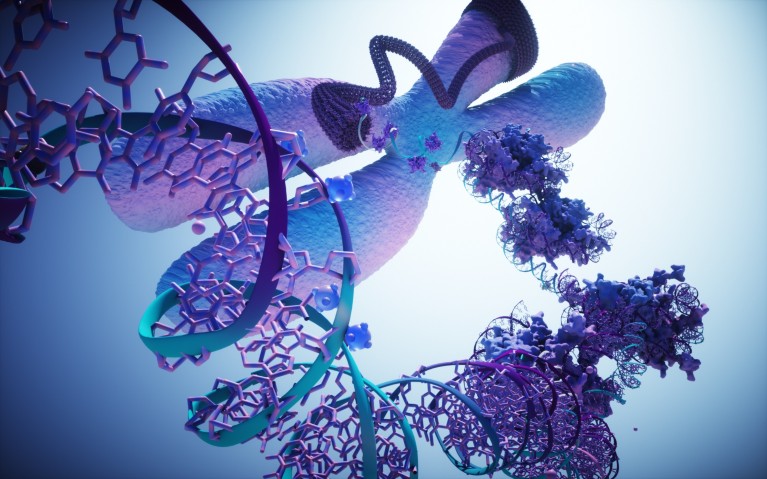
Epigenetic mechanisms affect how DNA is packaged into chromosomes, and hence how accessible the genes are for transcription.Credit: Ella Maru Studios
When it comes to cancer, mechanisms that affect the expression of genes without changing their coding sequence are as important as genetic mutations. However, research into these epigenetic processes lagged because they used to be hard to study. “New technologies are allowing us to catch up with genetics knowledge,” says Céline Vallot, a cancer epigeneticist at the Institut Curie in Paris.
Epigenetic processes typically work by changing the structure of chromatin: the DNA and histone proteins that make up chromosomes. If the chromatin is open and accessible, gene transcription can occur; where it’s more compact, the genes are silenced. Chromatin conformation can be altered by enzymes that chemically tag either the DNA or the histones it wraps around. Most epigenetic research has focused on processes that affect these enzymes, and how they might turn oncogenes on or tumour-suppressor genes off.
The structure of chromatin, and hence gene expression, is also affected by non-coding DNA, which makes up 98% of the genome. Notably, there are short regions that govern the expression of nearby genes, and attention is turning to mutations that affect these so-called enhancers and insulators. “Epigenetic alterations that change boundaries between gene neighbourhoods cause genes to come under inappropriate stimulation,” says Howard Chang, director of the Center for Personal Dynamic Regulomes at Stanford University, California. Chang’s group is pioneering methods to study the epigenome and identify key mechanisms regulating transcription in the fields of cancer, development and ageing.
The aberrant transcriptional profiles that result from epigenetic alterations can be used to not only distinguish different cancer subtypes, but also as a way to detect tumours from blood samples. Drugs that target epigenetic regulators are already approved for the treatment of myelodysplastic syndrome, T cell lymphomas and multiple myeloma.
However, epigenetic therapies are yet to realize their full potential. Current therapies are limited by toxicity and off-target effects. Advances in next-generation sequencing technologies are allowing researchers to map epigenomic information and its effects on chromatin structure in more detail than ever before. By studying the epigenetic landscape of cancer cells and the immune cells they do battle with, researchers are identifying new epigenetic targets and treatment approaches. This knowledge is helping to both improve the selectivity of epigenetic therapies and tailor treatments to the characteristics of each patient.
Epigenetics and immuno-oncology
Paediatric oncologist Crystal Mackall and her team at Stanford University are using ATAC-seq (Assay for Transposase-Accessible Chromatin using sequencing) to reveal epigenetic mechanisms regulating T-cell exhaustion, a state of progressive dysfunction that develops when the cells are continuously stimulated by antigens and inflammatory factors. Overcoming T-cell exhaustion is crucial if the durability of chimeric antigen receptor (CAR) T cell therapies is to improve.
“ATAC-seq provides unparalleled visualization of the most transcriptionally active regions of the genome,” she says. Mackall’s team has used ATAC-seq to identify the transcription factors that are upregulated during CAR T cell exhaustion.
The aim of the Stanford University team is to disrupt the transcriptional programmes that drive exhaustion-associated gene expression. They have found one likely pathway: overexpression of the transcription factor c-Jun seems to prevent exhaustion and improve tumour control, paving the way for next-generation exhaustion-proof CAR-T therapies.1
By combining epigenetic knowledge with gene editing technologies such as CRISPR, Mackall expects to see more ‘epigenetic engineering’, that will target the non-coding regulatory regions of the genome. “Rather than completely remove the expression of exhaustion-associated genes, through epigenetic engineering we can fine-tune their expression and prevent cells from reacting in a certain way in a particular context,” she explains. “We’ve seen that it works, we still need to find out if it is useful.”
Vallot agrees that further improvements in epigenetic technologies are required before current findings are translated to patients. “The technology is starting to be applied to patient samples,” she says. “I hope, in five years, it can be used to characterize large patient cohorts for personalized therapy.”
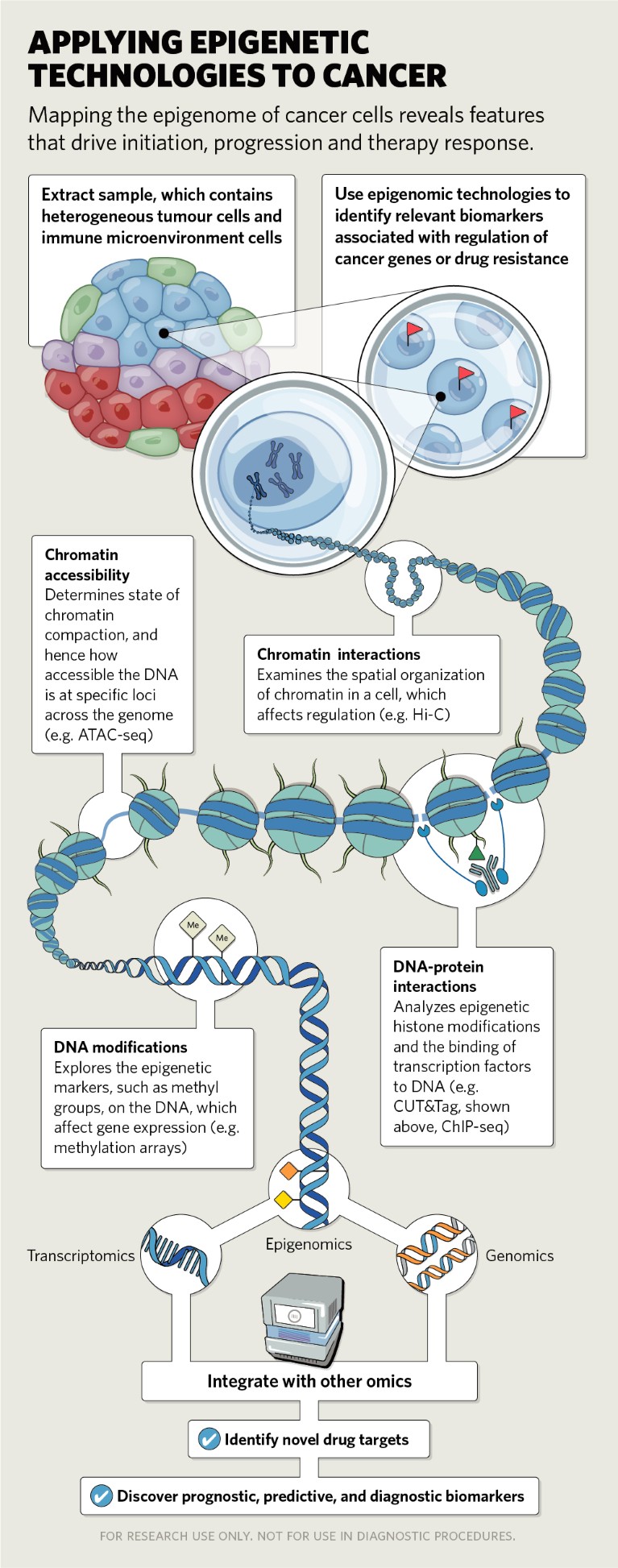
Emerging single-cell epigenetic technologies
Until recently, most studies have depended on techniques that measure average epigenetic states of bulk cell populations and tissues. Although they have provided invaluable information on generalized mechanisms, they don’t allow the examination of epigenetic events occurring in poorly characterized cell populations and rare cell types within normal or tumour tissues.
Understanding cancer cell heterogeneity, both within and between tumours, can shed light on features that determine the cells’ metastatic potential and sensitivity to treatment. More than 30 single-cell epigenomic methods have been reported,2 which can be used to assess the structure of chromatin or DNA accessibility (such as scATAC-seq), the 3-D organization of chromosomes (scHI-c) and genome-wide histone and DNA modifications (scChIP-seq, scCUT&Tag) [see 'Applying epigenetic technologies to cancer'].
Developmental epigeneticist Melanie Eckersley-Maslin has been using single-cell technologies to investigate epigenetic remodelling and cell fate commitment during the early stages of embryonic development. She has now joined the Peter MacCallum Cancer Centre in Melbourne, Australia, where her team will apply these methods to examine cancer cell-fate trajectories.
It’s well established that cancer cells can develop gene expression patterns that resemble those observed in embryonic cells, but researchers know little about how or why it happens. “It’s like cells have a mid-life crisis,” says Eckersley-Maslin, “they lose their identity and become more like embryonic cells, able to acquire different cell fates and adapt to new environments.” Scientists suspect that understanding the functional significance of these changes has the potential to reveal new cancer biomarkers and drug targets.
Researchers at the Institut Curie have developed an approach that involves single-cell chromatin immunoprecipitation followed by sequencing (scChIP-seq) to explore breast cancer cell plasticity and its implications for treatment. Using patient-derived xenograft models of breast cancer, they found a subset of untreated drug-sensitive cells that share a common chromatin signature with tamoxifen-resistant cells.3 Their work highlights the loss of a particular chromatin mark, which is associated with stable transcriptional repression (H3K27me3) as a key mechanism underlying drug resistance.
“Single cell approaches are paving the way to assess epigenetic heterogeneity within tumours, which is instrumental to understand cancer cell plasticity and the evolution process towards resistance,” says Vallot, the study’s lead author. She is focusing on how the cells change their epigenetic predisposition to become resistant to treatment over time, and whether chemotherapy can be combined with epigenetic modulators to prevent chemoresistance.4 “The timing is crucial,” she says. “Epigenetic modulators need to be provided at the onset of chemotherapy to restrict cancer cell plasticity and stop the evolution of resistance.”
An alternative method to investigate the genomic localization of histone modifications and transcription factors is Cleavage Under Targets and Tagmentation (CUT&Tag) technology.5 The starting material for CUT&Tag is live permeabilized cells or isolated nuclei, rather than cells or tissue that are crosslinked with formaldehyde and sheared, as is the case with ChIP. With CUT&Tag, the chromatin retrieved at the final step of the protocol is in the local vicinity of the binding sites of the protein of interest. The shorter DNA sequences isolated by CUT&Tag allow for a lower sequencing depth (3-5 million reads).
“CUT&Tag is an efficient way of profiling the epigenome of single cells and identifying the early stages of disease,” says drosophila-specialized geneticist Kami Ahmad, who has been developing the technology with Steven Henikoff at the Fred Hutchinson Cancer Research Center in Seattle, US. His team is applying high-throughput CUT&Tag to study both cancer biology in humans and developmental processes in fruit flies. Their latest work explores the epigenetic profile of single cells in samples from patients with mixed phenotype acute leukaemia.6 The chromatin heterogeneity among cells from the same patient could have major implications for treatment. “It seems that cells might interconvert between two epigenetic states — and treatments may drive epigenetic change,” he explains.
Putting it all together
Combining epigenomic techniques to profile multiple epigenetic marks within the same single cell and integrating them with transcriptional and genetic information will be key to generating comprehensive cell profiles and personalized cancer therapies. “By combining different technologies researchers are doing some really imaginative things and getting a better understanding of what is going on in cancer,” says Eckersley-Maslin.
Many multi-omics approaches are being developed to advance cancer research. They include technologies such as transcript-indexed ATAC-seq (T-ATAC-seq) that combine sequencing of the T cell receptor (TCR)-encoding genes with ATAC-seq to provide information on the TCR specificity and epigenomic state of individual T cells;7 Perturb-ATAC, which combines CRISPR perturbations with ATAC-seq to study relationships between factors that control chromatin states;8 or simultaneous high-throughput ATAC and RNA expression with sequencing (SHARE-seq), a highly scalable approach for measuring chromatin accessibility and gene expression in the same single cell.9
“The future is bright for advanced sequencing technology in cancer research,” says Chang.
“Multiomics and spatial genomic methods are two major advances that are helping us learn how to read and re-write the cancer genome.”