The CRISPR/Cas9 endonuclease allows the targeted disruption of genes across a wide range of cell lines and cell types. To this end, a single guide RNA (sgRNA) directs Cas9 to elicit a double-strand break at a genomic site that is complementary to the sgRNA. If the target sequence lies in a coding exon, this often leads to a frameshift mutation that disrupts the open reading frame. Delivery of multiple sgRNAs or entire sgRNA libraries allows unbiased functional genomic screens (so-called CRISPR screens). Initially, CRISPR screening had been confined to readouts such as cell proliferation or flow cytometry. More recently, the term “single-cell CRISPR screen” has been used for techniques from a series of landmark publications describing the combination of pooled CRISPR screens with single-cell RNA sequencing (referred to as Perturb-seq or CROP-seq)1,2,3,4. Phenotypic changes in global transcription of each cell are acquired by scRNA-seq and mapped back onto the causative sgRNA. The resulting matrix of sgRNAs versus the perturbed transcriptomes provides a strong dataset for the study of complex biological questions (Fig. 1). Here, a model experiment is described using scRNA-seq that compares an unbiased whole-transcriptome amplification (WTA) approach with an approach that nominates a panel of target mRNAs a priori.
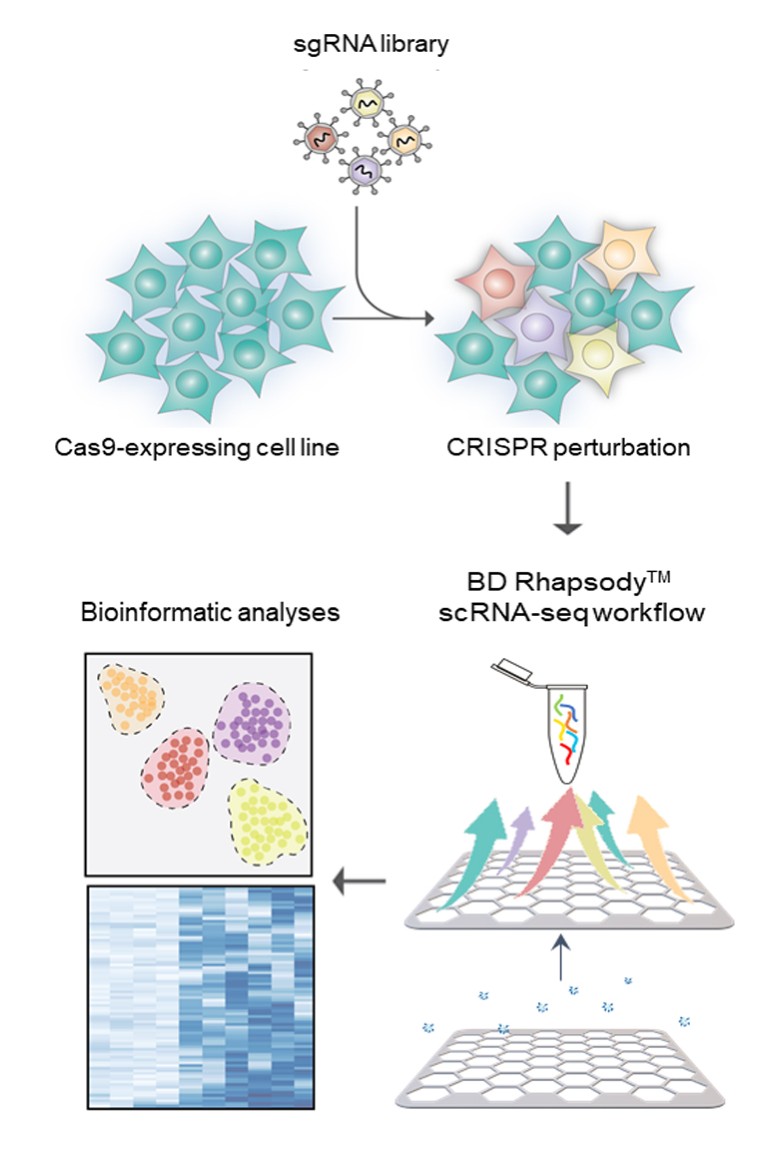
Figure 1 | Overview of a CRISPR single-cell screen. Cas9-expressing cells are transduced with a single guide RNA (sgRNA) library to perturb the expression of specific genes. Next, individual cells are isolated and the RNA-seq library is generated using the BD Rhapsody workflow. The impact of individual sgRNAs on the single cell’s transcriptome is then assessed by analysis of the RNA-seq data. Targeted sequencing offers a greater dynamic range at a more affordable price.
A targeted approach is affordable and more sensitive
Conventional single-cell RNA sequencing platforms have three major limitations: (i) high costs associated with single-cell library preparation and next-generation sequencing (NGS); (ii) limited sensitivity, as mRNAs of interest are often sparsely represented and outshone by abundant mRNAs from, for example, housekeeping genes; and (iii) lack of process control, as it is not possible to monitor metrics such as cell capture efficiency and multiplet rate, both of which strongly influence NGS data quality.
To illustrate the feasibility of targeted sequencing in conjunction with CRISPR screens, a single-cell RNA sequencing experiment was conducted using a WTA approach. Here, all expressed genes are amplified in a largely unbiased manner, resulting in a complex library ready for NGS. Analyzing such data reveals that the number of differentially expressed transcripts is typically low. However, only those genes that are differentially expressed across cells are considered informative, as they account for the cells’ specific phenotypes. Sequencing a complex WTA library consequently implies that many reads are spent on constitutively expressed mRNAs that are considered non-informative and thus cause unnecessary sequencing cost (Fig 2a).
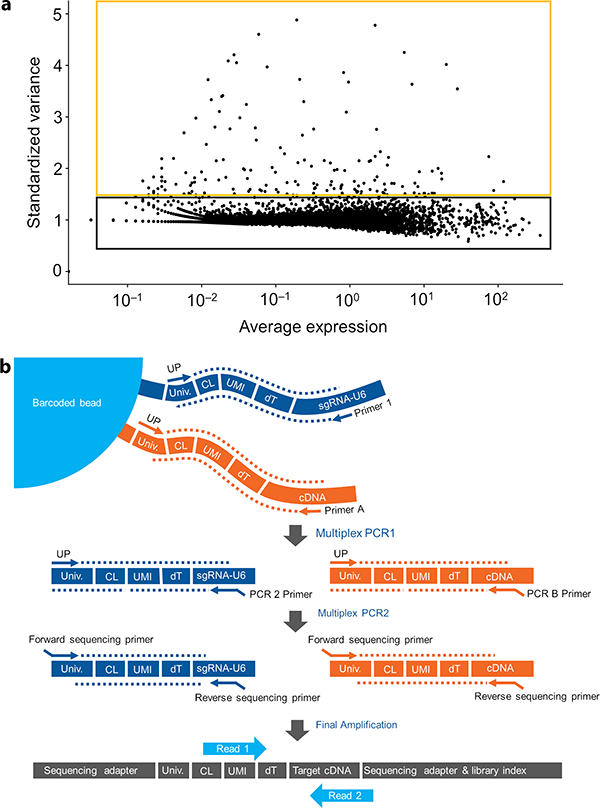
Figure 2 | Targeted single-cell mRNA-seq on the BD Rhapsody System. a, Highly dispersed genes are informative. Most scRNA-seq methods use a whole-transcriptome amplification (WTA) approach to generate a complex library representing all transcripts of that sample. On the basis of variance across cell types, transcripts can be segregated into non-informative and informative. The most variable transcripts are considered informative (orange frame) while stably and/or highly expressed ones are considered non-informative (black frame). Sequencing such a library results in low coverage of informative transcripts, as these are diluted by the non-informative ones. b, Targeted library preparation. mRNA molecules released from a lysed cell are captured on a magnetic bead via probes made of a universal primer binding site, a cell label, a unique molecular identifier (UMI) and a poly(dT) tail. After reverse transcription, the cDNA representing the transcriptome is covalently bound to the bead. Two multiplexed nested PCR reactions are performed using primers targeting both, the sgRNA and the genes of interest. Library preparation is completed by adding sequencing adaptors compatible with Illumina sequencing platforms. Read 1 reads through the cell label and UMI and read 2 reads into the cDNA insert.
The BD Rhapsody workflow provides an appealing solution to this dilemma, as it allows one to focus on a specific set of mRNAs that are selected prior to the experiment. First, single cells are individualized in microwells in a randomized, gravity-based manner and then combined with barcoded beads bearing an oligo(dT) primer. Following reverse transcription, the entire transcriptome of the cell is captured as cDNA on the surface of the beads. From there, the user has the option to run a WTA or a targeted assay (the WTA assay available on the BD Rhapsody System was not used in this study). For the targeted approach, only selected transcripts are amplified by two multiplexed nested PCR steps. This nested approach enhances specific amplification of the desired transcripts. Illumina adapters are added in a third PCR to finalize the targeted library ready for sequencing on any Illumina NGS platform (Fig 2b).
Overall, this process is highly flexible, as the transcripts of interest can be selected a priori by the user. Taking known cell-type-specific transcript variants into consideration, BD’s automated primer design pipeline assembles the panel of cognate primers, which can then be sourced through BD.
Perturbation of IFN signaling
To assess the feasibility of targeted sequencing, the JAK–STAT signaling pathway elicited by interferon-β (IFN-β) was used as a model. This pathway leads to specific transcriptional changes, and the cellular genes mediating signal transduction are well understood. For the CRISPR perturbation, a set of 27 genes was selected from the KEGG pathway database. This set consists of most of the cognate signaling intermediates (for example, STAT1 and STAT2) and was expanded to include other members of the same gene family. Human RKO cells harboring Cas9 were transduced with the sgRNA library and, nine days after transduction, cells were stimulated with IFN-β for 3 hours at 100 ng/mL and subjected to single-cell RNA sequencing. The experiment was set up to compare a conventional WTA approach to the above described targeted approach. The targeted sequencing experiment was focused on a set of 73 mRNAs. Roughly half of these were known to be interferon response genes; the other half were selected to represent the genes that were perturbed by CRISPR.
Targeted single-cell CRISPR screening
For single-cell separation, 22,400 cells were loaded onto the BD Rhapsody microwell cartridge and the BD Rhapsody scanner reported a multiplet rate of 5.3%. This is in close agreement with the theoretical multiplet rate of 5.2% predicted by the Poisson distribution model. 19,303 beads coupled to at least one cell were retrieved from the cartridge, which gives a capture rate of 86%. To enable a valid comparison of technologies, the retrieved beads were subsampled to 10,000 cells by volume pipetting, in line with the 10,000 cells used for the WTA approach.
While the WTA experiment was sequenced at a depth of 300 million reads, the targeted library was sequenced at 150 million reads, corresponding to 15,000 reads per cell. Out of the 73 transcripts in the targeted gene panel, 72 were detected in total. The median number of transcripts expressed per cell was 52 across the 10,000 cells studied. The sequencing saturation of this targeted library was 93%, indicating that a lower sequencing depth would have been sufficient. To further examine the correlation of sequencing depth and detection sensitivity, the dataset was bioinformatically subsampled and the representation of the 72 transcripts across all cells was plotted. For detection of most genes, it was concluded that even as few as 3,200 reads per cell are sufficient without losing much representation (Fig. 3a). This suggests that targeted experiments can be performed at 10% of the read depth of WTA experiments without sacrificing quality, which is supported by published data5.
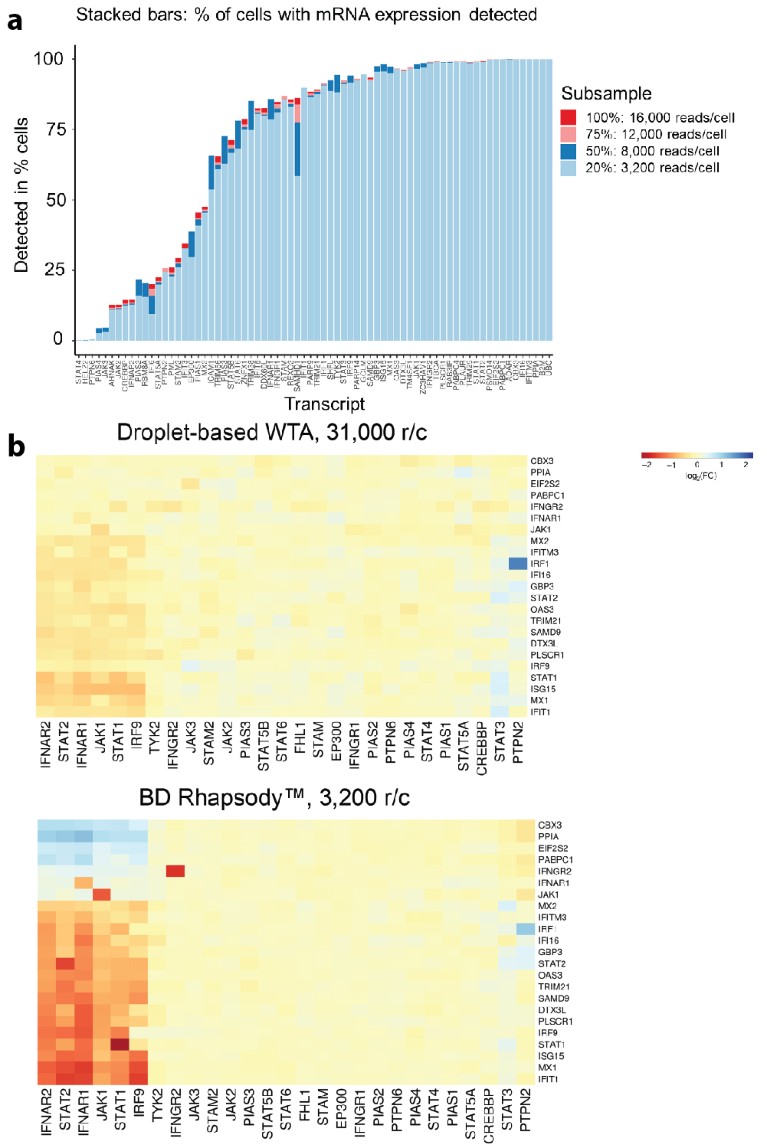
Figure 3 | A targeted single-cell mRNA-seq approach is cost-efficient while increasing sensitivity. a, Detection of targeted transcripts by NGS. A panel of 72 mRNAs containing many IFN response genes was selected for the targeted scRNA-seq approach. A median of 52 of the 72 mRNAs were detected per cell while 70 of 72 genes were detected in at least five cells. Subsampling the NGS data from 15,000 reads per cell (r/c) to 3,200 suggests that the representation of the mRNA signature can be maintained at lower read depth. b, Targeted sequencing offers greater dynamic range at lower NGS cost. Heat maps show fold changes (FC) of differentially expressed genes in log2 space. For better comparability, the range is set to –4 (red) and 4 (blue) in both plots. Whole-transcriptome amplification (WTA) data (above) and targeted amplification data (below). Focusing only on preselected mRNAs leads to a greater dynamic range of detection.
To assess the impact of CRISPR perturbations on the phenotype of the interferon signaling pathway, sgRNAs were mapped back onto cells. This is greatly facilitated by the targeted workflow, as the primer panel also includes a primer specific to the sgRNA scaffold. 77% of the cells contained an sgRNA. Cells bearing no sgRNA (12%) or more than one sgRNA (11%) were excluded from all further analyses. Figure 3b shows a heat map in which all sgRNAs targeting the same gene are aggregated and the logarithmic fold changes plotted. The x axis of this heat map shows the CRISPR perturbations while the y axis shows 23 transcripts that appear most informative out of the pool of 72 enriched transcripts. It is important to note that this experiment yielded distinct phenotypes for just six CRISPR perturbations: the two subunits of the interferon receptor (IFNAR1 and IFNAR2), a critical kinase downstream of the JAK1 receptor and a tripartite transcription factor complex (STAT1, STAT2 and IRF9). The remaining 21 perturbed genes did not seem to have an impact on the overall gene expression pattern of the cells studied, indicating that they do not play a role in this pathway. This observation is in agreement with the published literature. Even though the targeted library was only sequenced at about 10% of the number of reads used for the WTA library, a direct comparison reveals that the targeted sequencing approach provides a greater dynamic range.
Conclusion
This study showcases an efficient approach based on Aelian’s well-established CROP-seq workflow in combination with the targeted single-cell RNA sequencing assay on the BD Rhapsody Single-Cell Analysis System. Targeted sequencing lowers the cost and at the same time enhances the sensitivity of the experiment, enabling the detection of more subtle transcriptomic phenotypes. While single-cell CRISPR screens based on the WTA approach were limited to a few hundred perturbations, the targeted sequencing workflow presented here will allow genome-scale CRISPR screens.
Contact details and description
Aelian Biotechnology combines CRISPR screening with single-cell RNA sequencing, leveraging two transformative technologies to enable genetic screening for complex phenotypes. The CRISPR screening workflow can map the impact of thousands of genetic perturbations on the global transcriptome at single-cell resolution, thus effectively establishing a paradigm for next-generation CRISPR screens. The approach has broad applications in identifying novel drug targets or elucidating unknown mechanisms of actions of drugs.
If you are interested in working with Aelian, please contact an application specialist at info@aelianbio.com.
For further information on the BD Rhapsody™ System, please contact our applications team at scomix@bdscomix.bd.com.
Visit our community page for access to tech notes, brochures and demo data: https://scomix.bd.com.
BD Multi-Omics website: https://www.bdbiosciences.com/en-eu/applications/research-applications/multi-omics.