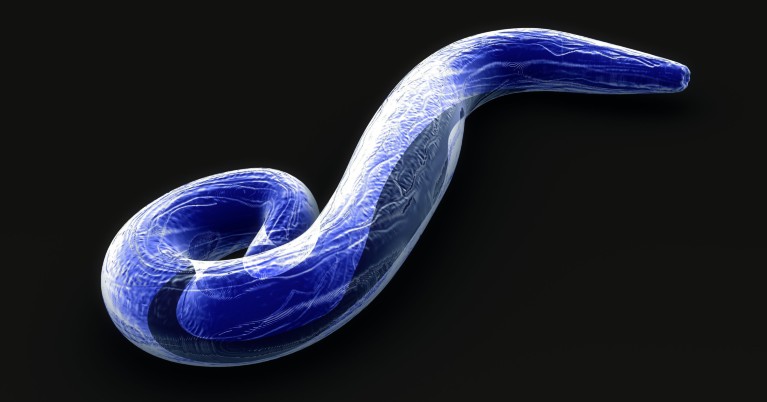
Illustration of a Plasmodium falciparum parasite. Credit: sciencepics/Shutterstock
The SARS-CoV-2 virus genome had barely been sequenced when there was a major effort to develop a safe and effective vaccine against it. But scientists working on COVID vaccines have one key thing in their favour: SARS-CoV-2 is a relatively simple pathogen.
The malaria parasite, Plasmodium falciparum, however, is an entirely more challenging pathogen because it is such a large and complex organism compared to a virus. At the Proteo-Science Center at Ehime University in Matsuyama, Japan, researchers have been using a new technology to study the thousands of proteins that make up the malaria parasite in an effort to identify the best targets for a new malaria vaccine.
P. falciparum is introduced into the body via a mosquito bite. The parasite grows and multiplies first in the liver, then uses human red blood cells as a nursery in which to replicate and release new parasites to invade other red blood cells. Malaria infection ranges in severity from asymptomatic to fatal disease, particularly if the parasites affect the brain.
To develop a vaccine for malaria, scientists must find a clear protein signature in P. falciparum, known as the antigen, that will be recognized and attacked by a host’s immune system. To find the most promising antigens from among thousands of malaria proteins, scientists created recombinant proteins. These proteins are made by introducing genes from a target organism — in this case, P. falciparum — into the genetic code of another organism, such as E. coli bacterium. That organism then transcribes and then translates those malaria parasite genes into P. falciparum proteins, so scientists can learn more about the proteins’ structure and function.
The common bacterium E. coli is traditionally used to produce recombinant proteins, but it has quite a low success rate, says Eizo Takashima, a protein biochemist from the Division of Malaria Research at the Proteo-Science Center.
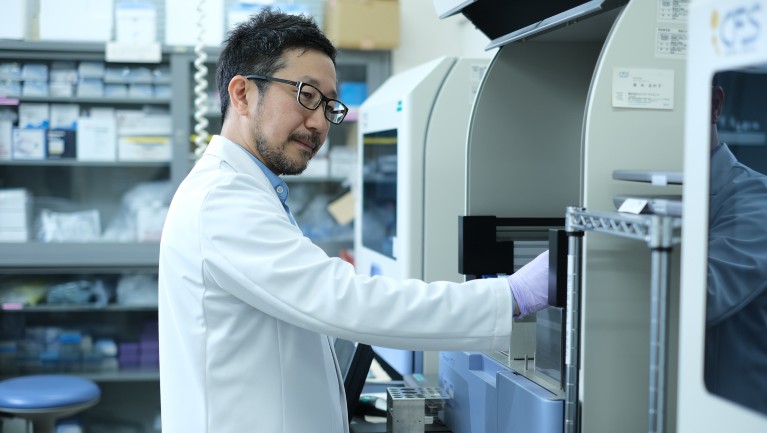
Eizo Takashima operating Protemist DT II, a robotic automatic protein synthesizer at Ehime University.
THE GOOD KIND OF GERM
Twenty years ago, Tatsuya Sawasaki, from the Division of Cell-Free Sciences at the Proteo-Science Center, used wheat germ as an alternative to E. coli for the production of recombinant proteins. This is the part of wheat that is normally removed when the grain is processed into flour and other wheat products. Sawasaki discovered that wheat germ contained all the cellular machinery needed to express proteins, and could do so more effectively and efficiently than E. coli. He then used that knowledge to develop the wheat germ cell-free system for expressing recombinant proteins, which is incorporated into technology produced by Ehime-spinoff company CellFree Sciences, such as the GenDecoder 1000 and Protemist DT II, which are able to generate large volumes of high-quality recombinant proteins.
The genome of the malaria parasite, P. falciparum, was sequenced back in 2002, which revealed around 5,500 genes, compared to just 10 in SARS-CoV-2. But despite the availability of the malaria parasite’s genome, relatively little is known about the proteins produced by that genome. “We know the sequences of the genome of malaria, but we don’t know the function of the proteins,” Takashima says.
Takashima and colleagues are now using the wheat germ cell-free system to synthesize recombinant malaria proteins from the P. falciparum genome. The wheat germ cell-free system has features that make it particularly well suited to producing recombinant malaria parasite proteins. “The advantage of the wheat germ cell-free system is that it can produce natively folded proteins without an artificial sugar chain, which is perfectly matched with malaria parasite protein features,” Takashima says. “The success rate is quite high, so we have production of more than 4,000 malaria proteins.”
With this new ability to produce malaria proteins, the researchers are able to use an approach called ‘reverse vaccinology’ to look for ones that will be best suited for a vaccine target. Firstly, they immunize rabbits and mice with some of the malaria proteins to look at what antibodies — the immune proteins that identify and bind to threats in the body — the rabbit and mice produce against those malaria proteins. They chose a group of malaria proteins that are key to the parasite’s ability to invade red blood cells, because these are proteins that are so important that they are unlikely to mutate in response to a threat such as a vaccine.
At the same time, they’ve been studying the blood of people who have previously been infected with malaria to identify the antibodies they have produced in response to their infection.
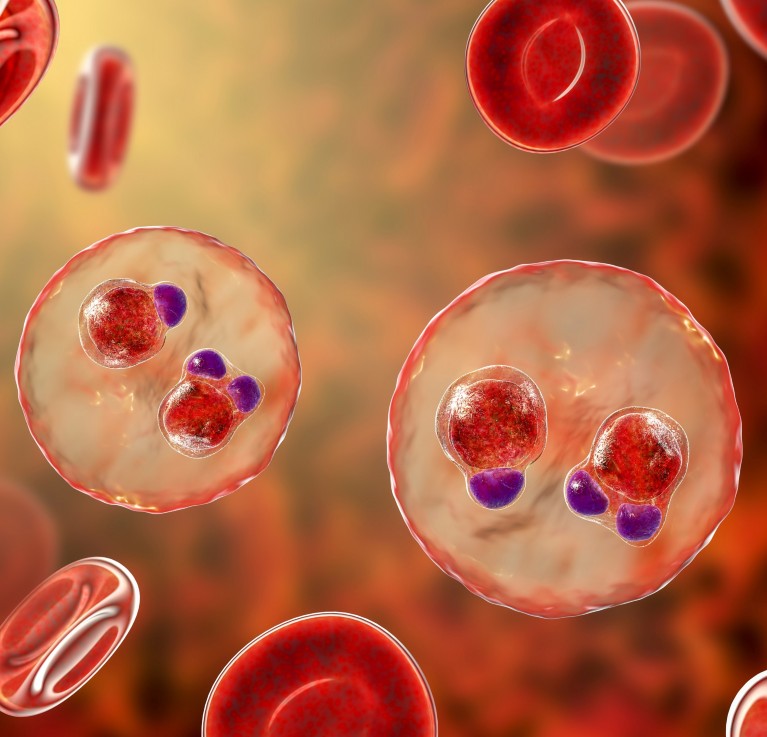
Illustrations of malaria-infected red blood cells. Credit: Kateryna Kon/Shutterstock
“Using the combination of the 4,000 malaria proteins and the samples taken from people in malaria-endemic areas, we can then profile the antibodies and see which malaria antigen is important for the protective effect,” Takashima says.
This approach has revealed one particular malaria antigen that could be useful, which was labelled PfRipr. However it’s an extremely large protein which would be difficult to manufacture. The next challenge was therefore to find a smaller segment of that protein that could be more easily produced but could still generate a protective immune response.
They have now singled out 11 small sections of that protein which, when injected into rats, produce antibodies that are effective at neutralizing the malaria parasite. Those smaller proteins are now being investigated as possible candidates to be developed into a vaccine.
LOOKING AHEAD
Malaria isn’t the only disease that the wheat germ cell-free system can help with. Sawasaki says the approach could be used to identify potential vaccine targets in a range of different diseases, from the mosquito-borne West Nile Virus to autoimmune diseases in which the immune system attacks healthy tissues.
For Takashima, the motivation for his work on malaria is to create a safer, healthier world. “My dream is that all people born on this planet would have the same chance to grow up safely.”